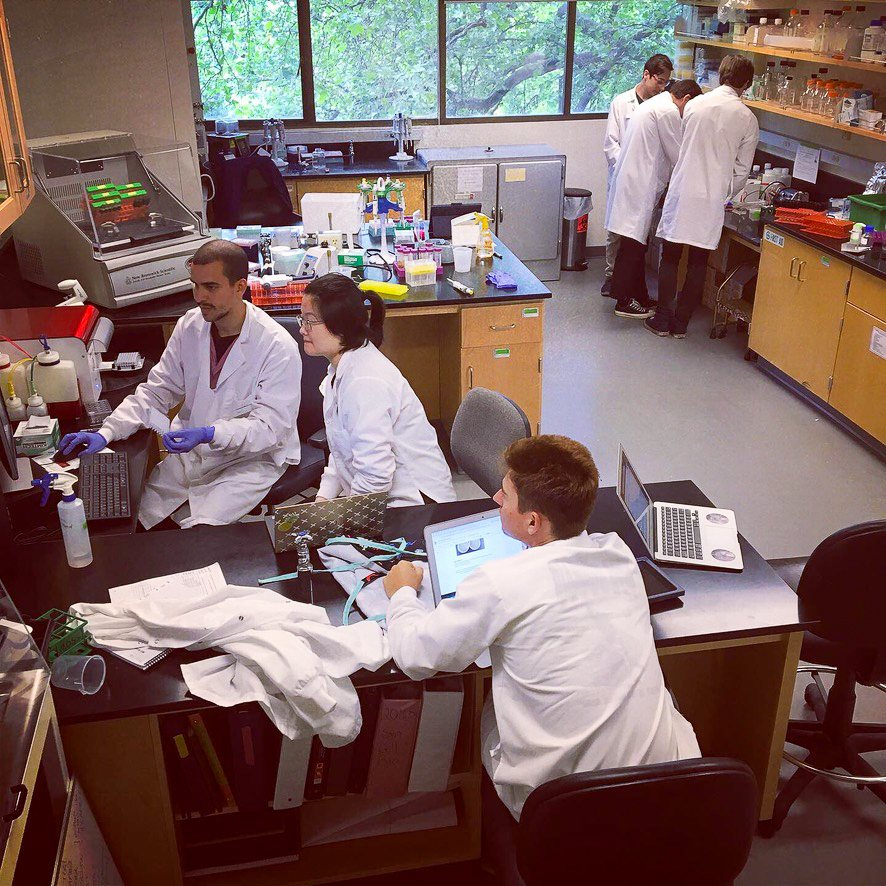
Plant-based burgers that bleed, microbes that produce unnaturally strong spider silk, face masks with a CRISPR sensor for wearable detection of SARS-CoV-2 and plants that can be programmed like computers: the products of synthetic biology research sometimes seem like gadgets sent from the future. Nonetheless, they are changing what we eat, how we heal, what we wear and how we take care of our environment.
In recent years, the field of synthetic biology has grown exponentially in part due to advances in tools like DNA sequencing and genome editing. Its applications span the areas of medicine, agriculture, food science, the environment and materials, drawing the interest of not just life science researchers, but engineers, technology think-tanks and private and public investors.
Synthetic biology uses engineering principles to design living systems that solve human problems. Taking a design-build-test approach, it differs from other biology subdisciplines that follow the traditional scientific method of drawing hypotheses from observation of natural systems. Instead of learning from observation to manipulate and engineer, synthetic biologists build to learn.
Engineering organisms’ genetic material to have new characteristics is a key feature of synthetic biology. It is being used in the field of medicine to produce novel medicinal compounds with designer cells or increase the yields of natural products, creating genetic circuits for tumor targeting and even re-engineering an injection structure found in bacteria to deliver customizable proteins into cells which could help us overcome the challenge of cell-specific drug delivery.
New discoveries stemming from the growth of synthetic biology as a field are also being used in the realm of agriculture and the environment to engineer organisms to use carbon dioxide, produce biofuels for vehicles and transform methane into biodegradable plastics. It is also being used to engineer crops that can produce higher yields in increasingly harsh growing conditions, which may be the key to our ability to continue growing food in a changing climate.
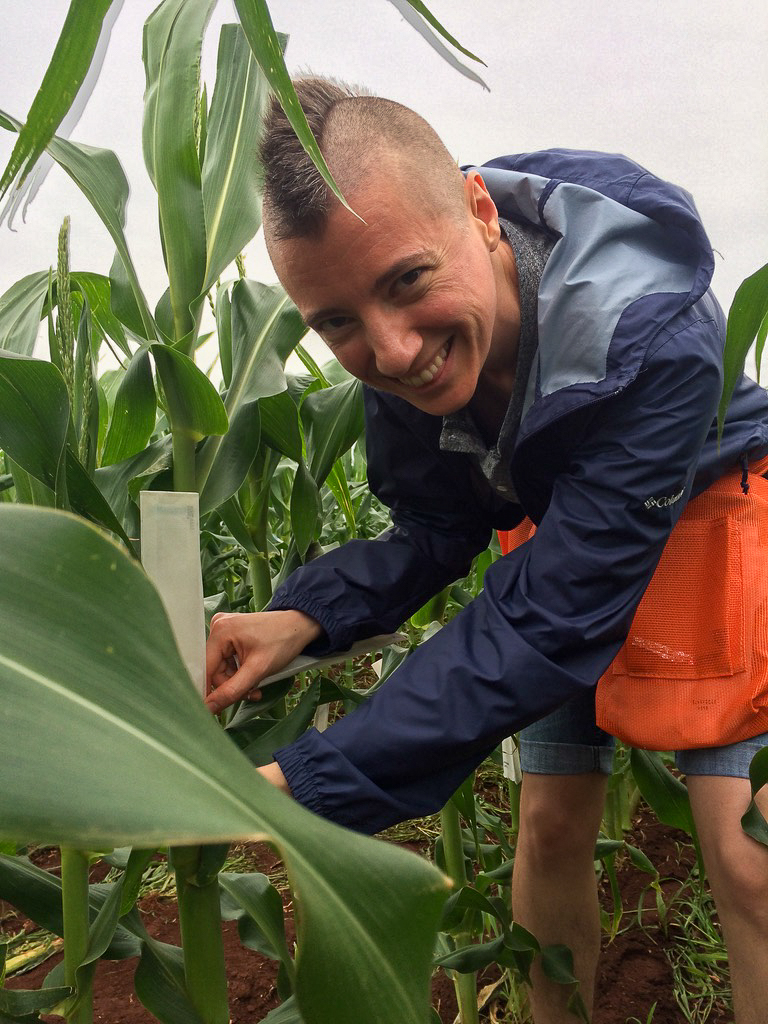
The challenge of controlling plant growth is the central research focus of Associate Professor of Biology, Biochemistry, Biophysics and Molecular Biology Brit Moss. She studies a hormone called auxin that choreographs many plant growth characteristics. Like a conductor, auxin orchestrates the growth patterns in different parts of plants. Have you noticed how your houseplant grows towards the window? That is auxin controlling phototropism (growth towards light). How does a plant know to grow its roots downwards? Auxin directs gravitropism in response to the abundance of water or nutrients.
Auxin is an especially interesting hormone because it is involved in almost every aspect of plant biology. It is essential for root growth, stalk growth, fruit development and determining stem and root branching. Traditional methods of developing crops take a long time. Understanding and eventually being able to quickly control how plants grow under certain conditions could be a crucial part of climate change adaptation and ensuring sufficient and equitable access to food.
“[The] ultimate goal of this kind of work is really to be able to adapt crops for specific environmental conditions … to basically be able to breed or engineer crop plants, for example, that are drought resistant, that might be heat tolerant, might be flood tolerant,” Moss said. “As the climate is rapidly changing, we need to equally as rapidly be able to adapt our crops in the face of the different ways the climate is impacting different parts of the world.”
During undergrad and grad school, Moss had focused on biomedical research in cancer cell biology, studying how chronic inflammation and cancer can be related. As she was finishing up her PhD, having stayed within one subfield for a decade, she was interested in going a different direction.
“I was going to talks on campus while I was a grad student, and I saw some really interesting talks from plant biologists who were doing fascinating types of research that wouldn’t be possible to do in humans or animals … using systems like microbes and plants that I wasn’t used to seeing,” Moss said.
This sparked Moss’s interest in combining parts of different organisms, and she eventually found a laboratory that was working specifically with synthetic biology.
“It was this group of plant and developmental biologists and electrical engineers and computer scientists who were trying to think about how we understand biology from a very different perspective … Can you apply engineering principles to understand biology and to eventually engineer it?” Moss said.
What had drawn me to the Biochemistry, Biophysics and Molecular Biology major was the realization that, despite how abstract it seemed as a first-year, this type of biology now feels to me like the study of machines. Incredibly complex, hard-to-understand machines, but machines all the same. When I think about biological systems on the molecular level, I am thinking about physical, visualizable cogs that make the wheel of life go ‘round.
These cogs are in the form of proteins, and the machine’s blueprint is the DNA. Together, proteins are like teams of small workers that carry out the instructions DNA gives them. Using engineering approaches, Moss creates different teams of these protein workers from plants and yeast to investigate and control how they function together and what physiological changes they can carry out in the plant. More specifically, Moss’ lab investigates the system that makes auxin able to control so many different types of plant characteristics: the three-part system that includes auxin, its receptor and a gene repressor.
Interestingly, instead of studying the auxin system directly in plants, Moss builds it up with its different components in bacteria and yeast. Using three organisms accelerates the building process.
Without auxin present, the repressor is like the brake pads on your bike, preventing the DNA from being read. When auxin comes into the picture, however, it binds the repressor to the receptor like glue, making the repressor unable to do its job.
“We think these repressors are actually like a tuning knob to kind of help time the response. And so you could imagine if you can alter it, you can basically tune that dial faster or slower, you’re going to impact that downstream response that’s happening,” Moss said.
Recent alumnus Andrew Methner ‘23 worked in Moss’s laboratory during his senior year when Moss was looking specifically at the auxin system in pear trees. The auxin pathway in pears has not been well studied – if better understood, it could be used as a model to understand similar pathways in similar crops like apples or cherries.
“It was kind of exciting to work in a particular lab where you’re doing something where you don’t know the results. Whether or not your hypothesis is accurate, you’re seeing what you want to see, or you’re seeing nothing, that’s all going to be the outcome of your work and it’s going to teach you something,” Methner said.
Senior Jake Wang worked with Moss last year investigating a different piece of the system: the receptor. By making mutations and changing the structure of the receptor protein, he was able to learn about its function. Wang emphasizes that as one of his first experiences with research, working in the Moss lab was eye-opening.
“It gave me a new perspective on what science is all about, and it has given me so many other opportunities … [I’ve learned] how to work in a lab, how to work with other people, how to learn new techniques, how to figure things out when they go wrong,” Wang said.
The findings from this research could lead to the development of programmable plants with tunable characteristics like depth of root growth.
“Eventually it could even be such that the farmer could actually tune and adjust [development] as needed during the growing season, depending on the weather. If, for example, there was some sort of chemical input that you could spray on the plant … the farmer could actually have sort of real-time control over some of these [characteristics],” Moss said.
But as Moss explains, the potential success of these “pie-in-the-sky” applications comes down to how well we understand the system we are trying to control, making basic research like Moss’s an essential part of the process.
“All of that really is predicated on basic biology research to understand … how development is controlled naturally in the plant. We need to understand that in many cases before we can start engineering it.”
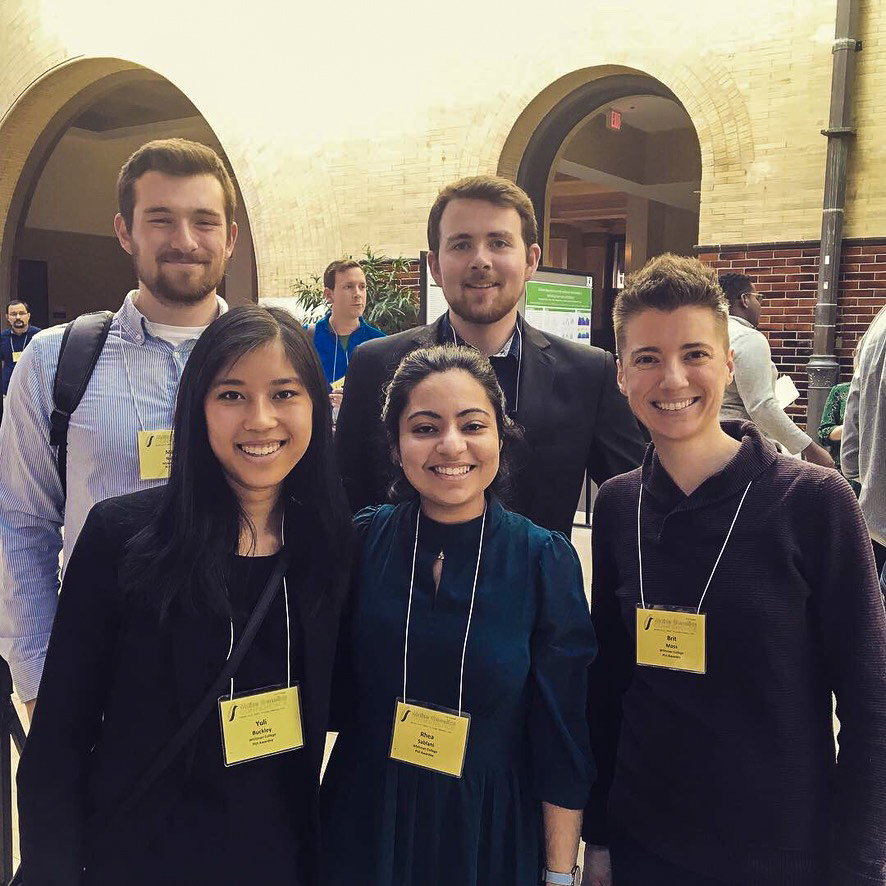